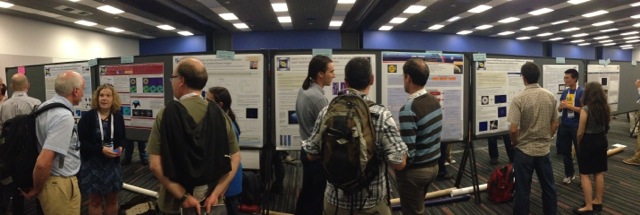
One of the walls of GPI-focused papers at #SPIEastro in Montreal on Monday June 23 (credit: M. Perrin)
Hello all,
It was an important week for the Gemini Planet Imager Consortium. Several of us met at SPIE Astro in Montreal, Quebec, Canada to present our work on GPI. Katie Morzinski wrote a blog post describing the GPI -focused events at the conference, so I will briefly give my perspective.
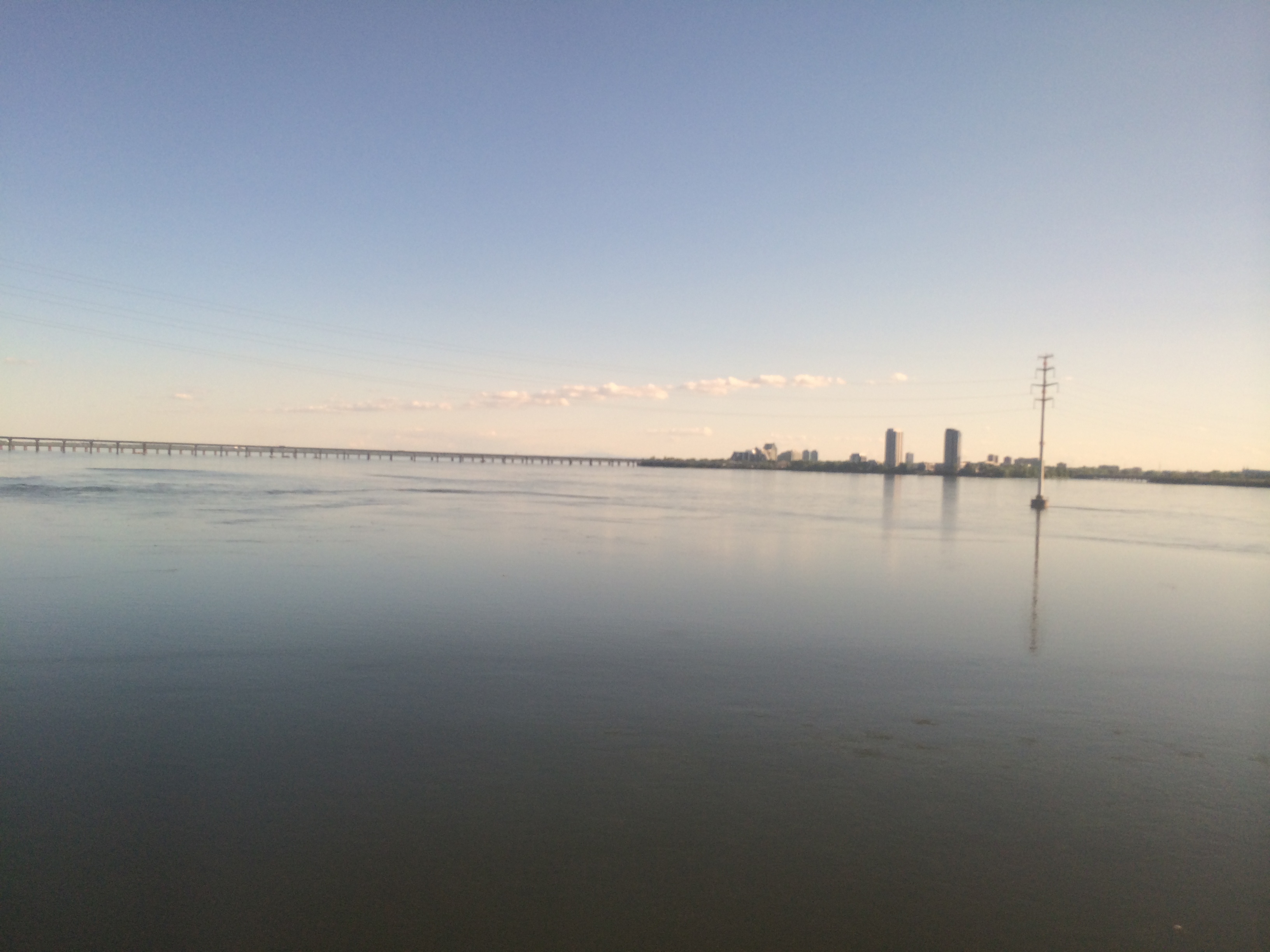
Arriving at Montreal by train. (credit: F. Marchis)
Yesterday, we submitted simultaneously 18 proceedings summarizing the analysis of data collected during the first light runs. I attached at the bottom of this post the complete list of proceedings. I recommend you check them if you want to know more about the first sky-results with this fantastic instrument.
The conference was also an opportunity for several members of the consortium to meet again, and for some of us to meet for the first time after several years communicating by emails. The Dunlap Institute, one of the partners of the consortium, invited the GPI team to a dinner in a fantastic Italian restaurant. Thanks Quinn for coordinating this memorable GPI event.
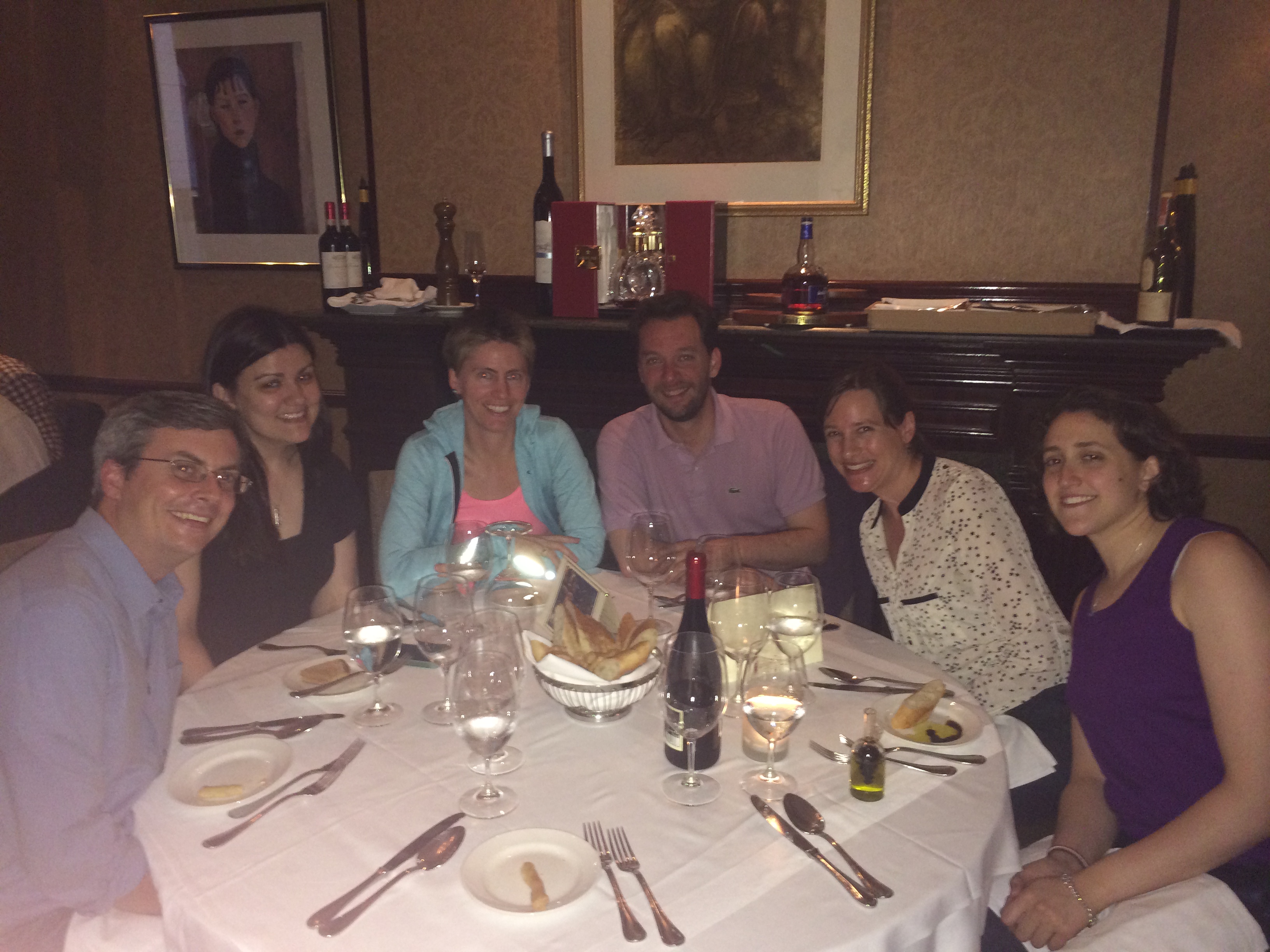
One of the GPI dinner tables: : Mike Fitzgerald, Quinn Konopacky, Lisa Poyneer, Laurent Pueyo, Katie Morzinski and Alexandra Greenbaum (credit: F. Marchis)
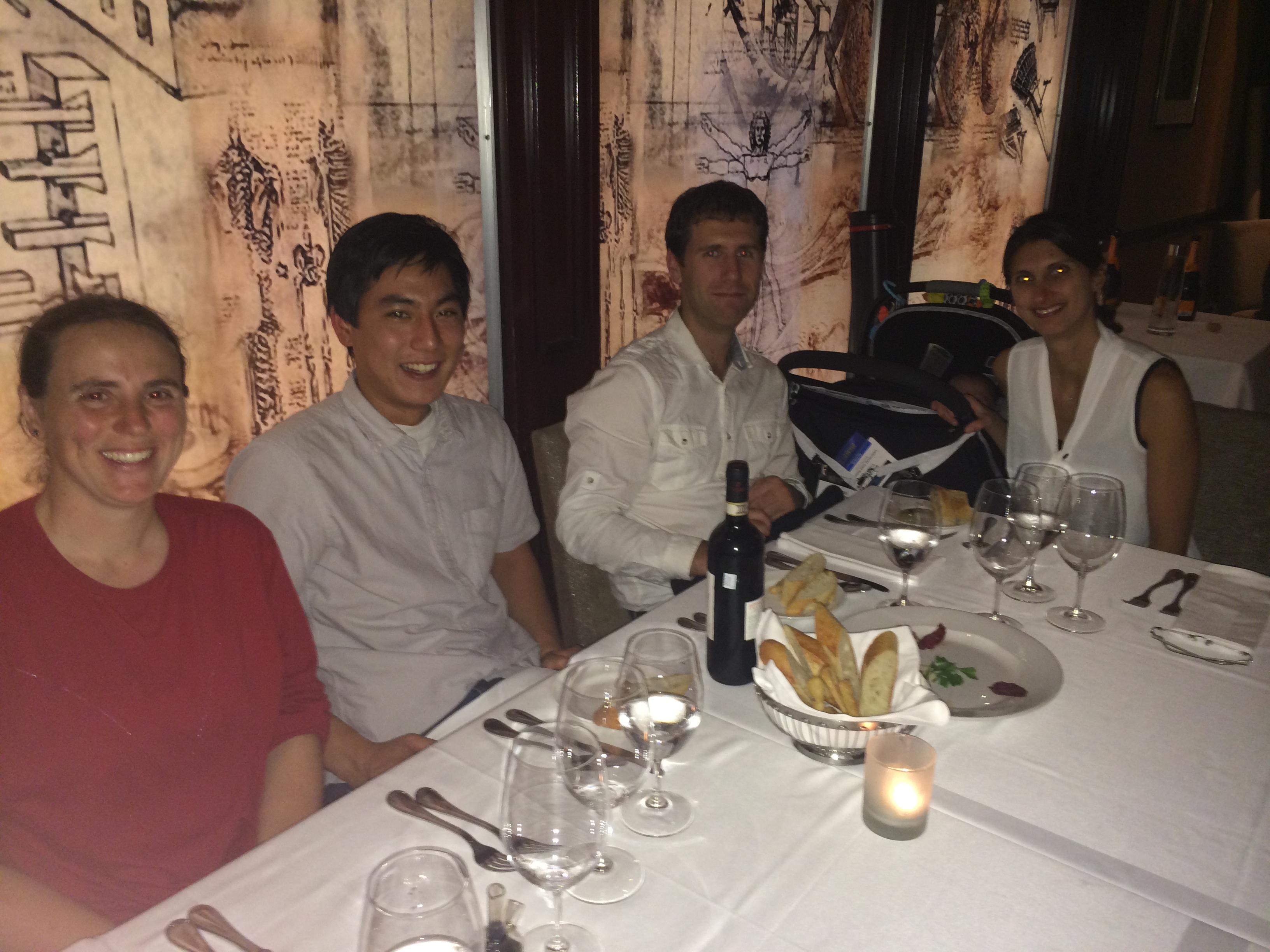
One of the GPI dinner tables: Pascale Hibon, Naru Sadakuni, Patrick Ingraham, Sandrine Thomas and Lyra, one of the youngest GPI members. (credit: F. Marchis)
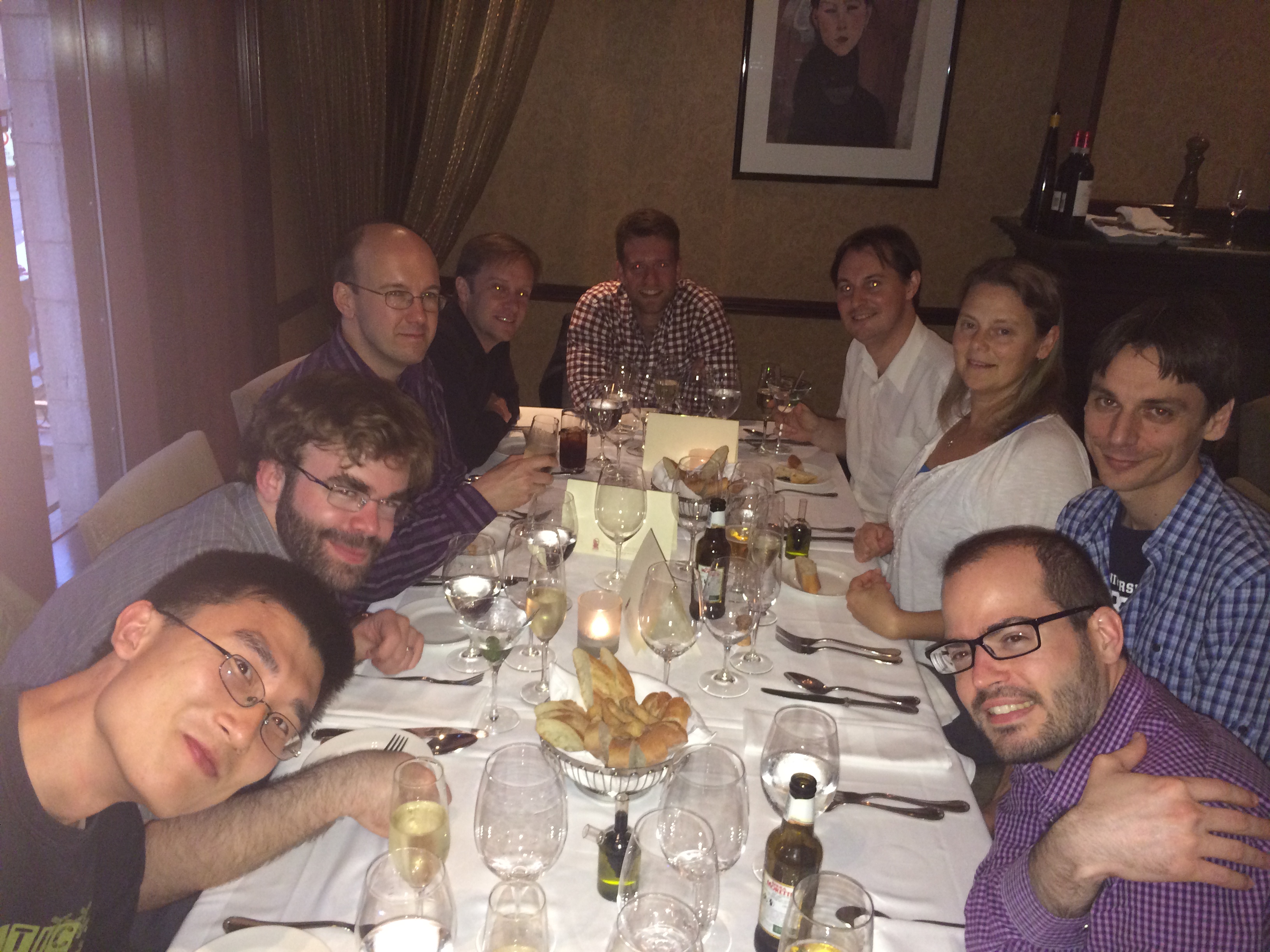
One of the GPI dinner tables: Jason Wang, Dmitri Savransky, Bruce Macintosh, James Larkin, Max Millar-Blanchaer, Marshall Perrin, Jennifer Dunn, Jérome Maire, Mark Ammons (credit: F. Marchis)
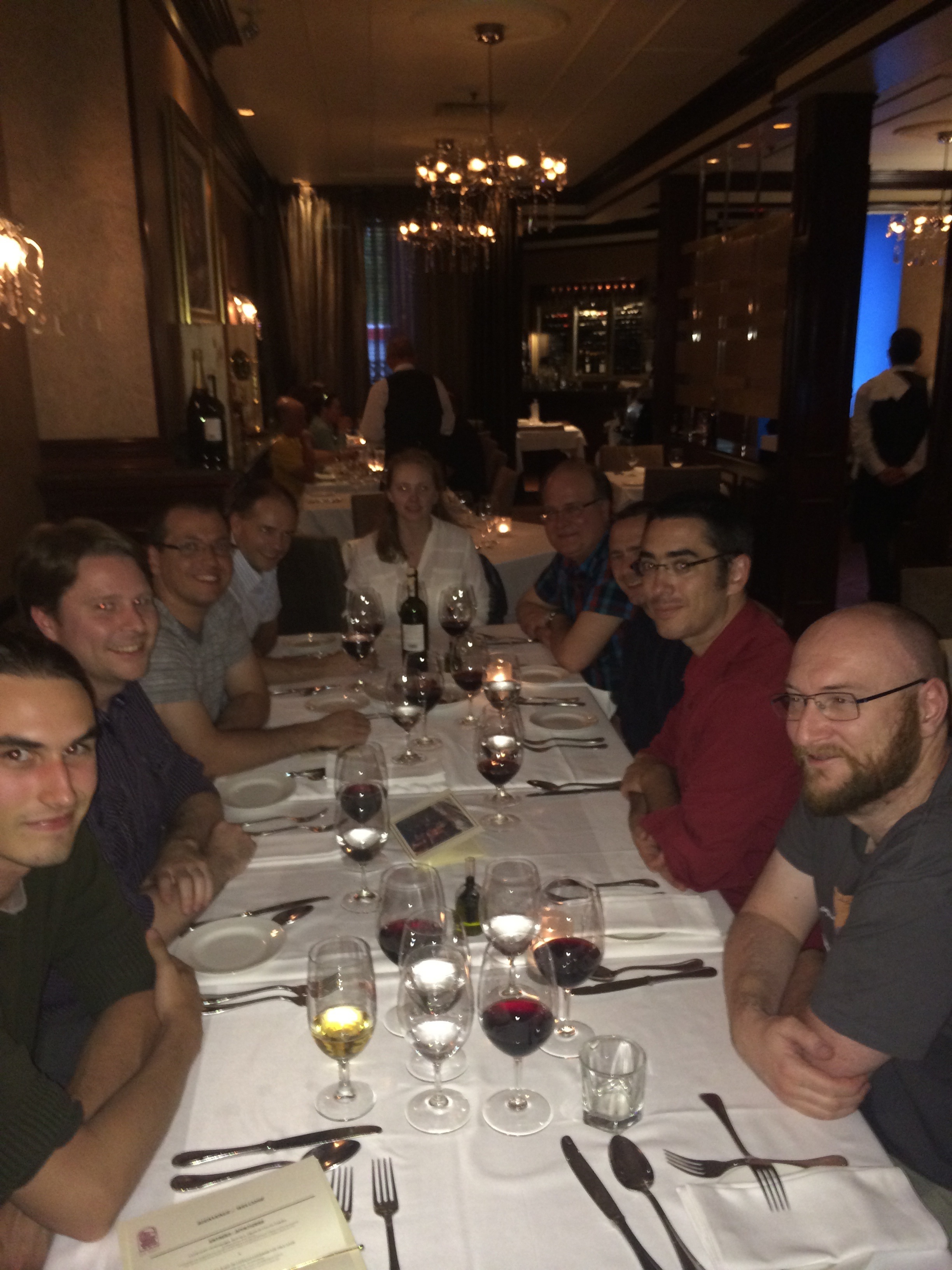
One of the GPI dinner tables: Dan Kerley, Zack Draper, Christian Marois, Markus Hartung, Schuyler Wolff, Fredrik, Stephen Goodsell, Etienne Artigau, Andrew Cardwell (credit: F. Marchis)
Thanks to the entire team for this great work.
See you in September at Chicago for the GPI Science meeting.
Clear skies,
Franck M.
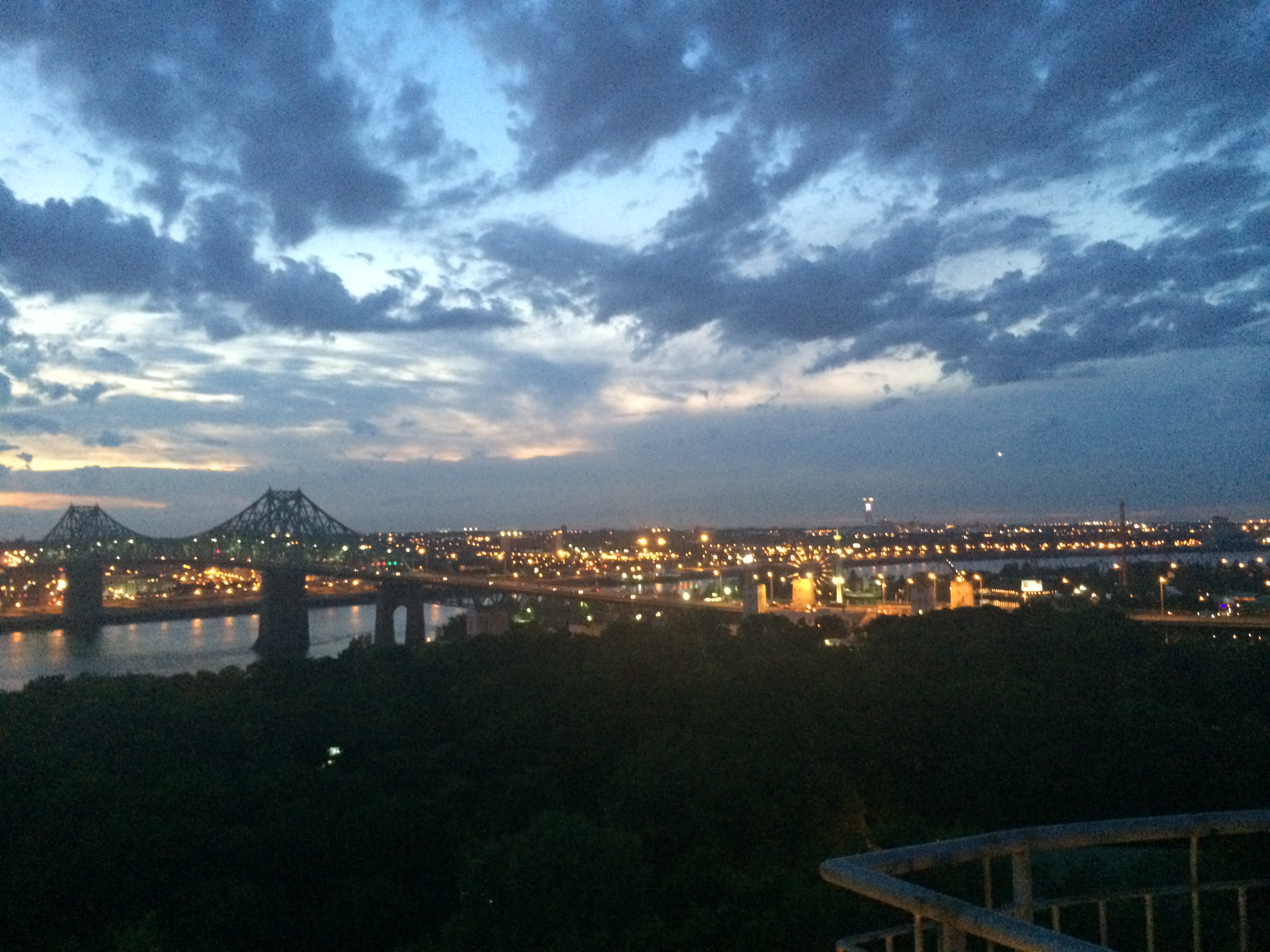
Sunset on Montreal (Credit:F. Marchis)
To directly image exoplanets and faint circumstellar disks, the noisy stellar halo must be suppressed to a high level. To achieve this feat, the angular differential imaging observing technique and the least-squares Locally Optimized Combination of Images (LOCI) algorithm have now become the standard in single band direct imaging observations and data reduction. With the development and commissioning of new high-order high-contrast adaptive optics equipped with integral field units, the image subtraction algorithm needs to be modified to allow the optimal use of polychromatic images, field-rotated images and archival data. A new algorithm, TLOCI (for Template LOCI), is designed to achieve this task by maximizing a companion signal-to-noise ratio instead of simply minimizing the noise as in the original LOCI algorithm. The TLOCI technique uses an input spectrum and template Point Spread Functions (PSFs, generated from unocculted and unsaturated stellar images) to optimize the reference image least-squares coefficients to minimize the planet self-subtraction, thus maximizing its throughput per wavelength, while simultaneously providing a maximum suppression of the speckle noise. The new algorithm has been developed using on-sky GPI data and has achieved impressive contrast. This paper presents the TLOCI algorithm, on-sky performance, and will discuss the challenges in recovering the planet spectrum with high fidelity.
The Gemini Planet Imager (GPI) is a complex optical system designed to directly detect the self-emission of young planets within two arcseconds of their host stars. After suppressing the starlight with an advanced AO system and apodized coronagraph, the dominant residual contamination in the focal plane are speckles from the atmosphere and optical surfaces. Since speckles are diffractive in nature their positions in the field are strongly wavelength dependent, while an actual companion planet will remain at fixed separation. By comparing multiple images at different wavelengths taken simultaneously, we can freeze the speckle pattern and extract the planet light adding an order of magnitude of contrast. To achieve a bandpass of 20%, sufficient to perform speckle suppression, and to observe the entire two arcsecond field of view at diffraction limited sampling, we designed and built an integral field spectrograph with extremely low wavefront error and almost no chromatic aberration. The spectrograph is fully cryogenic and operates in the wavelength range 1 to 2.4 microns with five selectable filters. A prism is used to produce a spectral resolution of 45 in the primary detection band and maintain high throughput. Based on the OSIRIS spectrograph at Keck, we selected to use a lenslet-based spectrograph to achieve an rms wavefront error of approximately 25 nm. Over 36,000 spectra are taken simultaneously and reassembled into image cubes that have roughly 192×192 spatial elements and contain between 11 and 20 spectral channels. The primary dispersion prism can be replaced with a Wollaston prism for dual polarization measurements. The spectrograph also has a pupil-viewing mode for alignment and calibration.
Characterization of the Atmospheric Dispersion Corrector of the Gemini Planet Imager
Pascale Hibon, Sandrine Thomas, Jennifer Dunn, Jenny Atwood, Les Saddlemyer, Naru Sadakuni, Stephen Goodsell, Bruce Macintosh, James Graham,Marshall Perrin, Fredrik Rantakyrö, Vincent Fesquet, Andrew Serio, Carlos Quiroz, Andrew Cardwell, Gaston Gausachs, Dmitry Savransky, Dan Kerley,Markus Hartung, Ramon Galvez, Kayla Hardie
An Atmospheric Dispersion Corrector (ADC) uses a double-prism arrangement to nullify the vertical chromatic dispersion introduced by the atmosphere at non-zero zenith distances. The ADC installed in the Gemini Planet Imager (GPI) was first tested in August 2012 while the instrument was in the laboratory. GPI was installed at the Gemini South telescope in August 2013 and first light occurred later that year on November 11th. In this paper, we give an overview of the characterizations and performance of this ADC unit obtained in the laboratory and on sky, as well as the structure of its control software.
Naru Sadakuni, Bruce A. Macintosh, David W. Palmer, Lisa A. Poyneer, Claire E. Max, Dmitry Savransky, Sandrine J. Thomas, Andrew Cardwell, Stephen Goodsell, Markus Hartung, Pascale Hibon,Fredrik Rantakyrö, Andrew Serio, with the GPI team
The Gemini Planet Imager (GPI) is a new facility, extreme adaptive optics (AO), coronagraphic instrument, currently being integrated onto the 8-meter Gemini South telescope, with the ultimate goal of directly imaging extrasolar planets. To achieve the contrast required for the desired science, it is necessary to quantify and mitigate wavefront error (WFE). A large source of potential static WFE arises from the primary AO wavefront sensor (WFS) detector’s use of multiple readout segments with independent signal chains including on-chip preamplifiers and external amplifiers. Temperature changes within GPI’s electronics cause drifts in readout segments’ bias levels, inducing an RMS WFE of 1.1 nm and 41.9 nm over 4.44 degrees Celsius, for magnitude 4 and 11 stars, respectively. With a goal of <2 nm of static WFE, these are significant enough to require remedial action. Simulations imply a requirement to take fresh WFS darks every 2 degrees Celsius of temperature change, for a magnitude 6 star; similarly, for a magnitude 7 star, every 1 degree Celsius of temperature change. For sufficiently dim stars, bias drifts exceed the signal, causing a large initial WFE, and the former periodic requirement practically becomes an instantaneous/continuous one, making the goal of <2 nm of static WFE very difficult for stars of magnitude 9 or fainter. In extreme cases, this can cause the AO loops to destabilize due to perceived nonphysical wavefronts, as some of the WFS’s Shack-Hartmann quadcells are split between multiple readout segments. Presented here is GPI’s AO WFS geometry, along with detailed steps in the simulation used to quantify bias drift related WFE, followed by laboratory and on sky results, and concluded with possible methods of remediation.
The Gemini Planet Imager (GPI) is a next-generation, facility instrument currently being commissioned at the Gemini South observatory. GPI combines an extreme adaptive optics system and integral field spectrograph (IFS) with an apodized-pupil Lyot coronagraph (APLC) producing an unprecedented capability for directly imaging and spectroscopically characterizing extrasolar planets. GPI’s operating goal of 10−7 contrast requires very precise alignments between the various elements of the coronagraph (two pupil masks and one focal plane mask) and active control of the beam path throughout the instrument. Here, we describe the techniques used to automatically align GPI and maintain the alignment throughout the course of science observations. We discuss the particular challenges of maintaining precision alignments on a Cassegrain mounted instrument and strategies that we have developed that allow GPI to achieve high contrast even in poor seeing conditions.
The Gemini Planet Imager (GPI) is an “extreme” adaptive optics coronagraph system that is now on the Gemini South telescope in Chile. This instrument is composed of three different systems that historically have been separate instruments. These systems are the extreme Adaptive Optics system, with deformable mirrors, including a high-order 64×64 element MEMS system; the Science Instrument, which is a near-infrared integral field spectrograph; and the Calibration system, a precision IR wavefront sensor that also holds key coronagraph components. Each system coordinates actions that require precise timing. The observatory is responsible for starting these actions and has typically done this asynchronously across independent systems. Despite this complexity we strived to provide an interface that is as close to a one-button approach as possible. This paper will describe the sequencing of these systems both internally and externally through the observatory.
The Gemini Planet Imager is an extreme AO instrument with an integral field spectrograph (IFS) operating in Y, J, H, and K bands. Both the Gemini telescope and the GPI instrument are very complex systems. Our goal is that the combined telescope and instrument system may be run by one observer operating the instrument, and one operator controlling the telescope and the acquisition of light to the instrument. This requires a smooth integration between the two systems and easily operated control interfaces. We discuss the definition of the software and hardware interfaces, their implementation and testing, and the integration of the instrument with the telescope environment.
The Gemini Planet Imager (GPI) Extreme Adaptive Optics Coronograph contains an interferometric mode: a 10-hole non-redundant mask (NRM) in its pupil wheel. GPI operates at Y,J,H, and K bands, using an integral field unit spectrograph (IFS) to obtain spectral data at every image pixel. NRM on GPI is capable of imaging with a half resolution element inner working angle at moderate contrast, probing the region behind the coronagraphic spot. The fine features of the NRM PSF can provide a reliable check on the plate scale, while also acting as an attenuator for spectral standard calibrators that would otherwise saturate the full pupil. NRM commissioning data provides details about wavefront error in the optics as well as operations of adaptive optics control without pointing control from the calibration system. We compare lab and on-sky results to evaluate systematic instrument properties and examine the stability data in consecutive exposures. We discuss early on-sky performance, comparing images from integration and tests with the first on-sky images, and demonstrate resolving a known binary. We discuss the status of NRM and implications for future science with this mode.
The Gemini Planet Imager (GPI) is an instrument designed to directly image planets and circumstellar disks from 0.9 to 2.5 microns (the YJHK infrared bands) using high contrast adaptive optics with a lenslet-based integral field spectrograph. We develop an extraction algorithm based on a least-squares method to disentangle the spectra and systematic noise contributions simultaneously. We utilize two approaches to adjust for the effect of flexure of the GPI optics which move the position of light incident on the detector. The first method is to iterate the extraction to achieve minimum residual and the second is to cross-correlate the detector image with a model image in iterative extraction steps to determine an offset. Thus far, this process has made clear qualitative improvements to the cube extraction by reducing the Moir\'{e} pattern. There are also improvements to the automated routines for finding flexure offsets which are reliable to with ∼0.5 pixel accuracy compared to pixel accuracy prior. Further testing and optimization will follow before implementation into the GPI pipeline.
The Gemini Planet Imager (GPI) combines extreme adaptive optics, an integral field spectrograph, and a high performance coronagraph to directly image extrasolar planets in the near-infrared. Because the coronagraph blocks most of the light from the star, it prevents the properties of the host star from being measured directly. Instead, satellite spots, which are created by diffraction from a square grid in the pupil plane, can be used to locate the star and extract its spectrum. We describe the techniques implemented into the GPI Data Reduction Pipeline to measure the properties of the satellite spots and discuss the precision of the reconstructed astrometry and spectrophotometry of the occulted star. We find the astrometric precision of the satellite spots in an H-band datacube to be 0.05 pixels and is best when individual satellite spots have a signal to noise ratio (SNR) of >20. In regards to satellite spot spectrophotometry, we find that the total flux from the satellite spots is stable to ∼7% and scales linearly with central star brightness and that the shape of the satellite spot spectrum varies on the 2% level.
We present on-sky polarimetric observations with the Gemini Planet Imager (GPI) obtained at straight Cassegrain focus on the Gemini South 8-m telescope. Observations of polarimetric calibrator stars, ranging from nearly unpolarized to strongly polarized, enable determination of the combined telescope and instrumental polarization. We find the conversion of Stokes I to linear and circular instrumental polarization in the instrument frame to be I→(QIP,UIP,PIP,VIP)=(−0.037±0.010%,+0.4338±0.0075%,0.4354±0.0075%,−6.64±0.56%). Such precise measurement of instrumental polarization enables ∼0.1% absolute accuracy in measurements of linear polarization, which together with GPI’s high contrast will allow GPI to explore scattered light from circumstellar disk in unprecedented detail, conduct observations of a range of other astronomical bodies, and potentially even study polarized thermal emission from young exoplanets. Observations of unpolarized standard stars also let us quantify how well GPI’s differential polarimetry mode can suppress the stellar PSF halo. We show that GPI polarimetry achieves cancellation of unpolarized starlight by factors of 100-200, reaching the photon noise limit for sensitivity to circumstellar scattered light for all but the smallest separations at which the calibration for instrumental polarization currently sets the limit.
The Gemini Planet Imager (GPI) is a new facility instrument for the Gemini Observatory designed to provide direct detection and characterization of planets and debris disks around stars in the solar neighborhood. In addition to its extreme adaptive optics and corona graphic systems which give access to high angular resolution and high-contrast imaging capabilities, GPI contains an integral field spectrograph providing low resolution spectroscopy across five bands between 0.95 and 2.5 μm. This paper describes the sequence of processing steps required for the spectro-photometric calibration of GPI science data, and the necessary calibration files. Based on calibration observations of the white dwarf HD 8049B we estimate that the systematic error in spectra extracted from GPI observations is less than 5%. The flux ratio of the occulted star and fiducial satellite spots within coronagraphic GPI observations, required to estimate the magnitude difference between a target and any resolved companions, was measured in the H-band to be Δm=9.23±0.06 in laboratory measurements and Δm=9.39±0.11 using on-sky observations. Laboratory measurements for the Y, J, K1 and K2 filters are also presented. The total throughput of GPI, Gemini South and the atmosphere of the Earth was also measured in each photometric passband, with a typical throughput in H-band of 18% in the non-coronagraphic mode, with some variation observed over the six-month period for which observations were available. We also report ongoing development and improvement of the data cube extraction algorithm.
We present the results of both laboratory and on sky astrometric characterization of the Gemini Planet Imager (GPI). This characterization includes measurement of the pixel scale of the integral field spectrograph (IFS), the position of the detector with respect to north, and optical distortion. Two of these three quantities (pixel scale and distortion) were measured in the laboratory using two transparent grids of spots, one with a square pattern and the other with a random pattern. The pixel scale in the laboratory was also estimate using small movements of the artificial star unit (ASU) in the GPI adaptive optics system. On sky, the pixel scale and the north angle are determined using a number of known binary or multiple systems and Solar System objects, a subsample of which had concurrent measurements at Keck Observatory. Our current estimate of the GPI pixel scale is 14.14 ± 0.01 millarcseconds/pixel, and the north angle is -1.00 ± 0.03deg. Distortion is shown to be small, with an average positional residual of 0.26 pixels over the field of view, and is corrected using a 5th order polynomial. We also present results from Monte Carlo simulations of the GPI Exoplanet Survey (GPIES) assuming GPI achieves ~1 milliarcsecond relative astrometric precision. We find that with this precision, we will be able to constrain the eccentricities of all detected planets, and possibly determine the underlying eccentricity distribution of widely separated Jovians.
We present the wavelength calibration for the lenslet-based Integral Field Spectrograph (IFS) that serves as the science instrument for the Gemini Planet Imager (GPI). The GPI IFS features a 2.7″ x 2.7″ field of view and a 190 x 190 lenslet array (14.3 mas/lenslet) operating in Y, J, H, and K bands with spectral resolving power ranging from R ∼ 35 to 78. Due to variations across the field of view, a unique wavelength solution is determined for each lenslet characterized by a two-dimensional position, the spectral dispersion, and the rotation of the spectrum with respect to the detector axes. The four free parameters are fit using a constrained Levenberg-Marquardt least-squares minimization algorithm, which compares an individual lenslet’s arc lamp spectrum to a simulated arc lamp spectrum. This method enables measurement of spectral positions to better than 1/10th of a pixel on the GPI IFS detector using Gemini’s facility calibration lamp unit GCAL, improving spectral extraction accuracy compared to earlier approaches. Using such wavelength calibrations we have measured how internal flexure of the spectrograph with changing zenith angle shifts spectra on the detector. We describe the methods used to compensate for these shifts when assembling datacubes from on-sky observations using GPI.
The newly commissioned Gemini Planet Imager (GPI) combines extreme adaptive optics, an advanced coronagraph, precision wavefront control and a lenslet-based integral field spectrograph (IFS) to measure the spectra of young extrasolar giant planets between 0.9-2.5 um. Each GPI detector image, when in spectral model, consists of ~37,000 microspectra which are under or critically sampled in the spatial direction. This paper demonstrates how to obtain high-resolution microlens PSFs and discusses their use in enhancing the wavelength calibration, flexure compensation and spectral extraction. This method is generally applicable to any lenslet-based integral field spectrograph including proposed future instrument concepts for space missions.
The Gemini Planet Imager is a newly commissioned facility instrument designed to measure the near-infrared spectra of young extrasolar planets in the solar neighborhood and obtain imaging polarimetry of circumstellar disks. GPI’s science instrument is an integral field spectrograph that utilizes a HAWAII-2RG detector with a SIDECAR ASIC readout system. This paper describes the detector characterization and calibrations performed by the GPI Data Reduction Pipeline to compensate for effects including bad/hot/cold pixels, persistence, non-linearity, vibration induced microphonics and correlated read noise.
The Gemini Planet Imager (GPI) has as its science instrument an infrared integral field spectrograph/polarimeter (IFS). Integral field spectrographs are scientificially powerful but require sophisticated data reduction systems. For GPI to achieve its scientific goals of exoplanet and disk characterization, IFS data must be reconstructed into high quality astrometrically and photometrically accurate datacubes in both spectral and polarization modes, via flexible software that is usable by the broad Gemini community. The data reduction pipeline developed by the GPI instrument team to meet these needs is now publicly available following GPI’s commissioning.
This paper, the first of a series, provides a broad overview of GPI data reduction, summarizes key steps, and presents the overall software framework and implementation. Subsequent papers describe in more detail the algorithms necessary for calibrating GPI data. The GPI data reduction pipeline is open source, available from planetimager.org, and will continue to be enhanced throughout the life of the instrument. It implements an extensive suite of task primitives that can be assembled into reduction recipes to produce calibrated datasets ready for scientific analysis. Angular, spectral, and polarimetric differential imaging are supported. Graphical tools automate the production and editing of recipes, an integrated calibration database manages reference files, and an interactive data viewer customized for high contrast imaging allows for exploration and manipulation of data.
The Gemini Planet Imager instrument’s adaptive optics (AO) subsystem was designed specifically to facilitate high-contrast imaging. It features several new technologies, including computationally efficient wavefront reconstruction with the Fourier transform, modal gain optimization every 8 seconds, and the spatially filtered wavefront sensor. It also uses a Linear-Quadratic-Gaussian (LQG) controller (aka Kalman filter) for both pointing and focus. We present on-sky performance results from verification and commissioning runs from December 2013 through May 2014. The efficient reconstruction and modal gain optimization are working as designed. The LQG controllers effectively notch out vibrations. The spatial filter can remove aliases, but we typically use it oversized by about 60% due to stability problems.